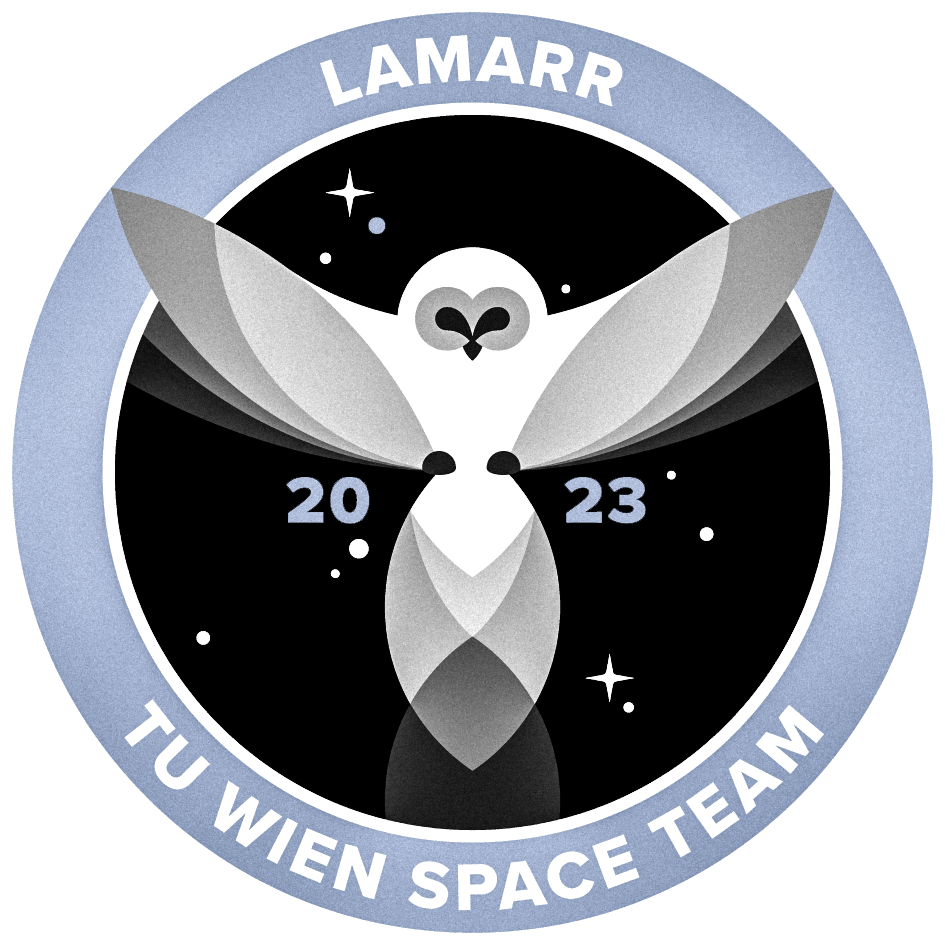
Lamarr
Project Lamarr is the continuation of our bi-liquid rocket project µHoubolt. Building on all the knowledge gained during µHoubolts development, project Lamarr plans to improve on our previous design with a more robust and reliable system reaching more ambitious goals with the rocket “Hedy”. The project and rocket name are chosen in memory of the Austrian actress and inventor Hedy Lamarr.
Mission objective and requirements
- Flight to an altitude of 9km
- Successful recovery with 2-stage parachute system
- Gathering telemetry and performance data throughout the full duration of the flight
- Thorough documentation to conserve knowledge in our team
In the early stages of our project, we decided to continue the mentality of light-weight design established by µHoubolt and build a rocket with an ambitious target weight of 25 kg, including all propellants. To achieve this, we aim for high system integration and the implementation of self developed solutions that focus on reliability and weight reduction. A perfect example for this philosophy are our structurally integrated propellant tanks, which maximize the weight efficiency of our tanks. To supply the necessary thrust needed for our target altitude, a new engine is being developed, manufactured and tested in-house to a large degree. A large effort has also been put into our new engine test stand, without which our testing procedures wouldn’t be possible.
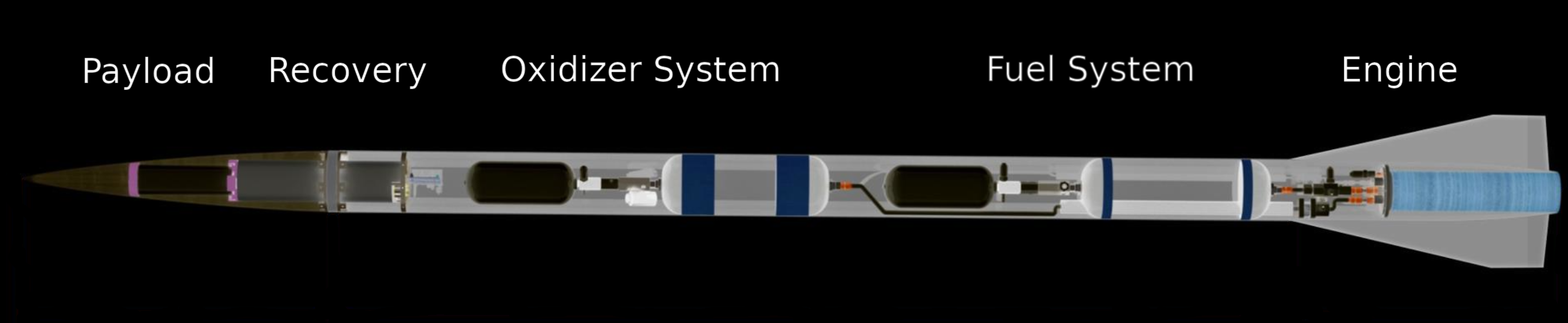
Propulsion
The engine runs on a fuel-rich Liquid Oxygen-Ethanol mixture, providing the necessary thrust of 2 kN. The feed system, which consists of various valves, pressure reducers and some safety features, supplies the engine with the required propellants and a pintle-type injector is being used to maintain a constant mass flow and achieve high atomization and mixture of both propellants in the combustion chamber. In order to manage the temperatures of over 2000°C in the engine, the combustion chamber is manufactured out of phenolic resin, acting as an ablative coolant.
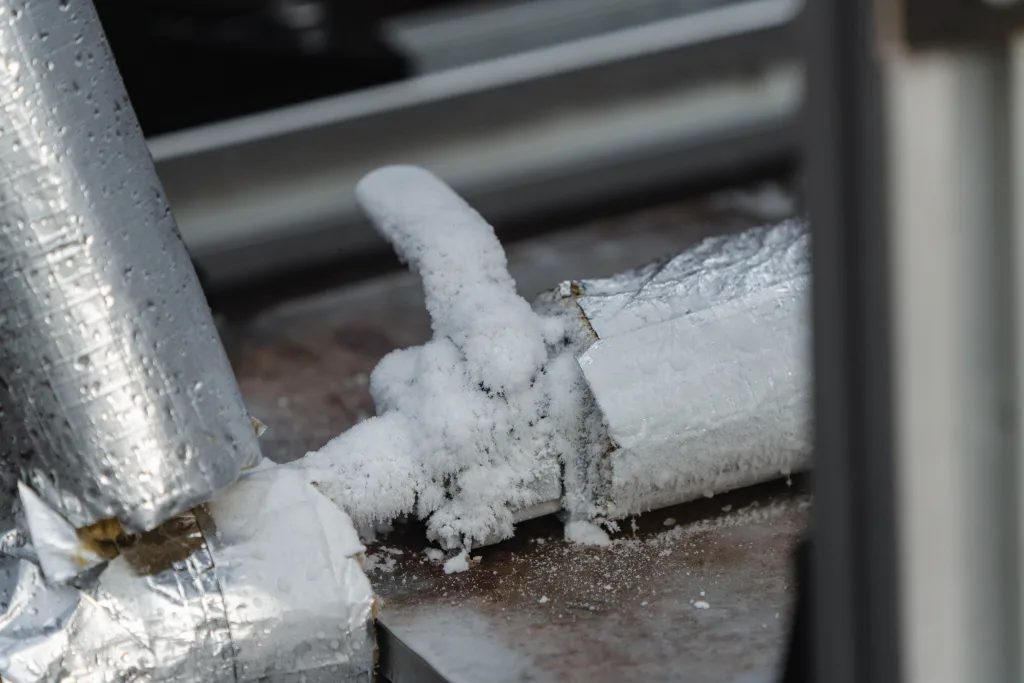
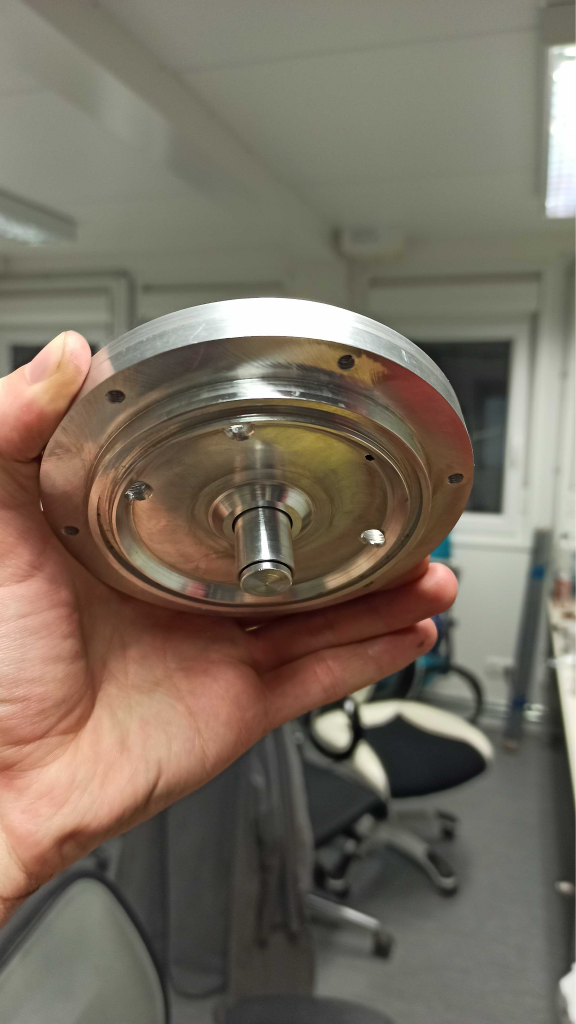
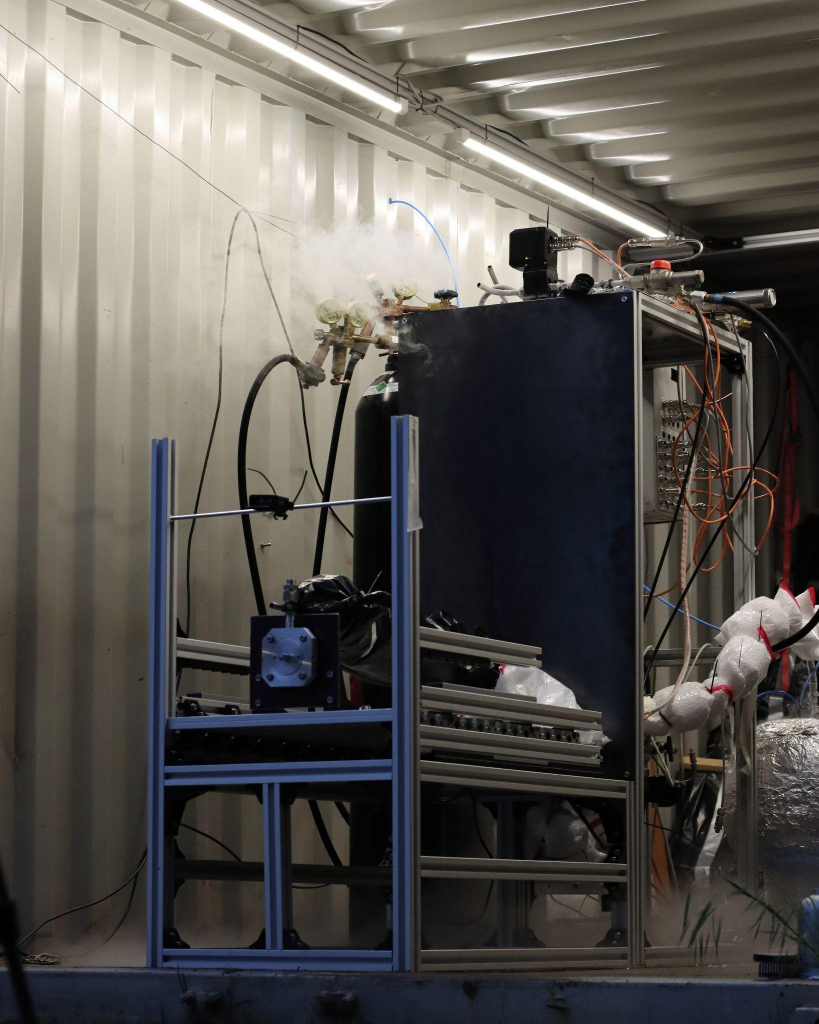
Aerostructure
Our rocket’s aerostructure comprises the nosecone, body tube, and fin can, with the oxidizer and fuel tanks designed as structural components, sharing the body tube’s outer diameter. The body tube has an inner diameter of 130 mm, with a wall thickness of 2.1 mm at its thickest point near the oxidizer tank, reduced to 1.6 mm outside of the tank area for weight reduction. The oxidizer tank’s bonding presents challenges due to low temperature and adhesive compatibility with liquid oxygen, which required extensive testing of different adhesives and procedures. The tank is subjected to pressurization tests and CT scans to check for irregularities like air entrapments. Insulation is applied to prevent ice buildup and reduce boiloff. Mass distribution favors stability, with the upper tank for liquid oxygen and the lower tank for ethanol. The nosecone shape, determined via parametric CFD study, is based on the Von Karman shape for optimal performance. Made from fiberglass and tempered epoxy resin, the nosecone withstands temperatures of up to 150°C, meeting the challenges posed by supersonic velocities.
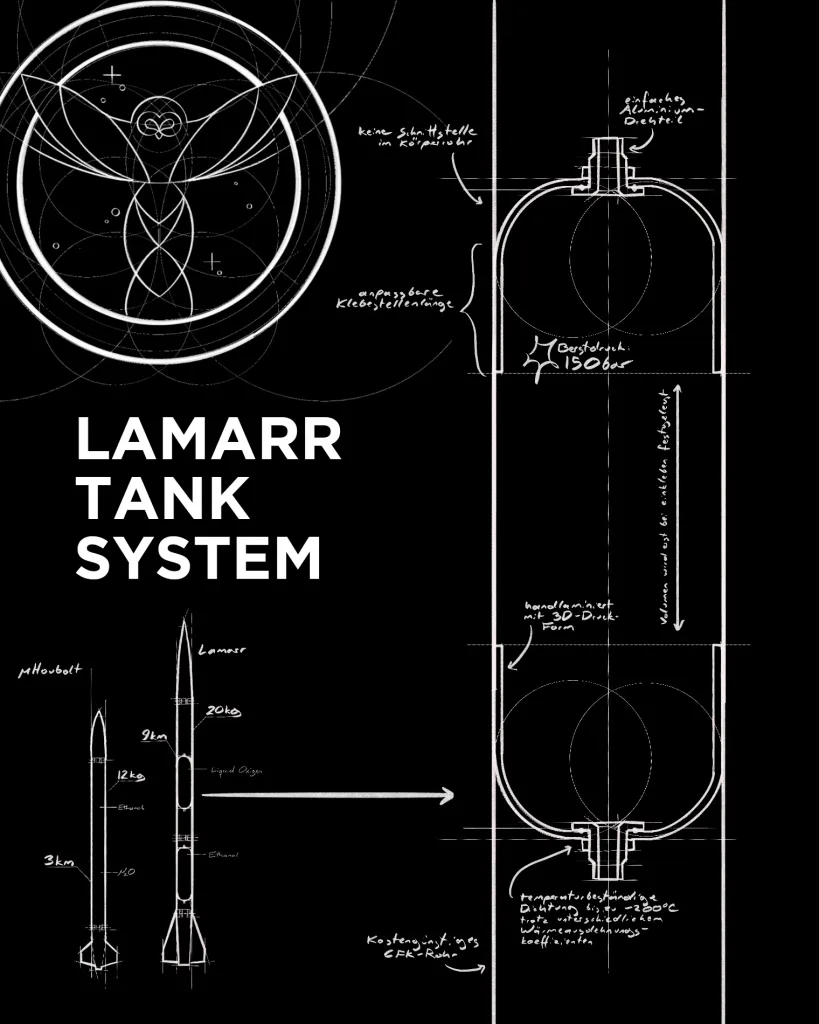
Recovery
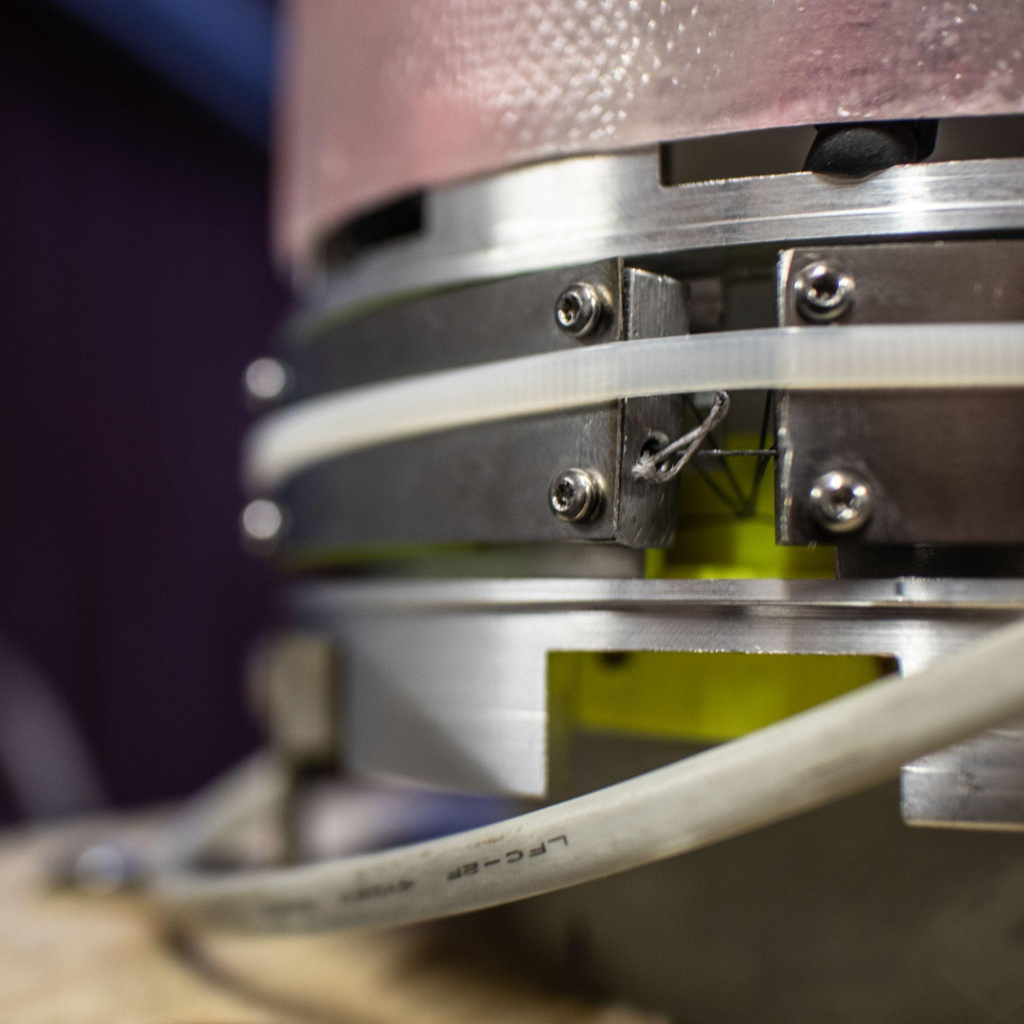
The recovery system features a two-stage parachute deployment, with a drogue parachute deployed at apogee and the main parachute deployed about 500m above ground. The drogue parachute, designed as a round cap, ensures a stable descent with a velocity of around 25 m/s. Deployment is triggered by redundant Altimax flight computers. Separation of the nosecone from the main body tube at apogee is achieved using a clamp band mechanism, minimizing overlap and complexity while eliminating the need for moving parts or motors. To reduce costs and allow for more preflight tests, an SRAD burn wire replaces expensive single-use line cutters. The main parachute is deployed by releasing the drogue parachute, ensuring a controlled descent with a velocity of around 6 m/s. Ongoing improvements focus on enhancing quality, reliability, and usability, with flight testing planned using a mock-up rocket flying to 700m.
Avionics
Project Lamarr’s avionics is developed in-house to a large extent andbuilds on lessons from the predecessor project μHoubolt, involving multiple modules communicating via a CAN bus interface. Power is managed by multiple Power Management Units (PMUs), with various battery systems and charging circuitry included. An umbilical on the launch rail supplies power and enables communication with Ground Support Equipment (GSE) via the bus during launch preparations. Telemetry data, including GNSS, barometric, IMU, and CAN messages, is collected and transmitted to the ground station via LoRa by the Radio Control Unit (RCU) for propulsion system evaluation before recovery. The custom Engine Control Unit (ECU) is key, controlling propellant valve and sensors, along with electronic pressure regulators. A brushless DC motor with a special FOC Controller is utilized for operating the ox main valve, using a COTS STMicroelectronics DevKit for its compact size. Adapter PCBs and custom connectors facilitate component integration.
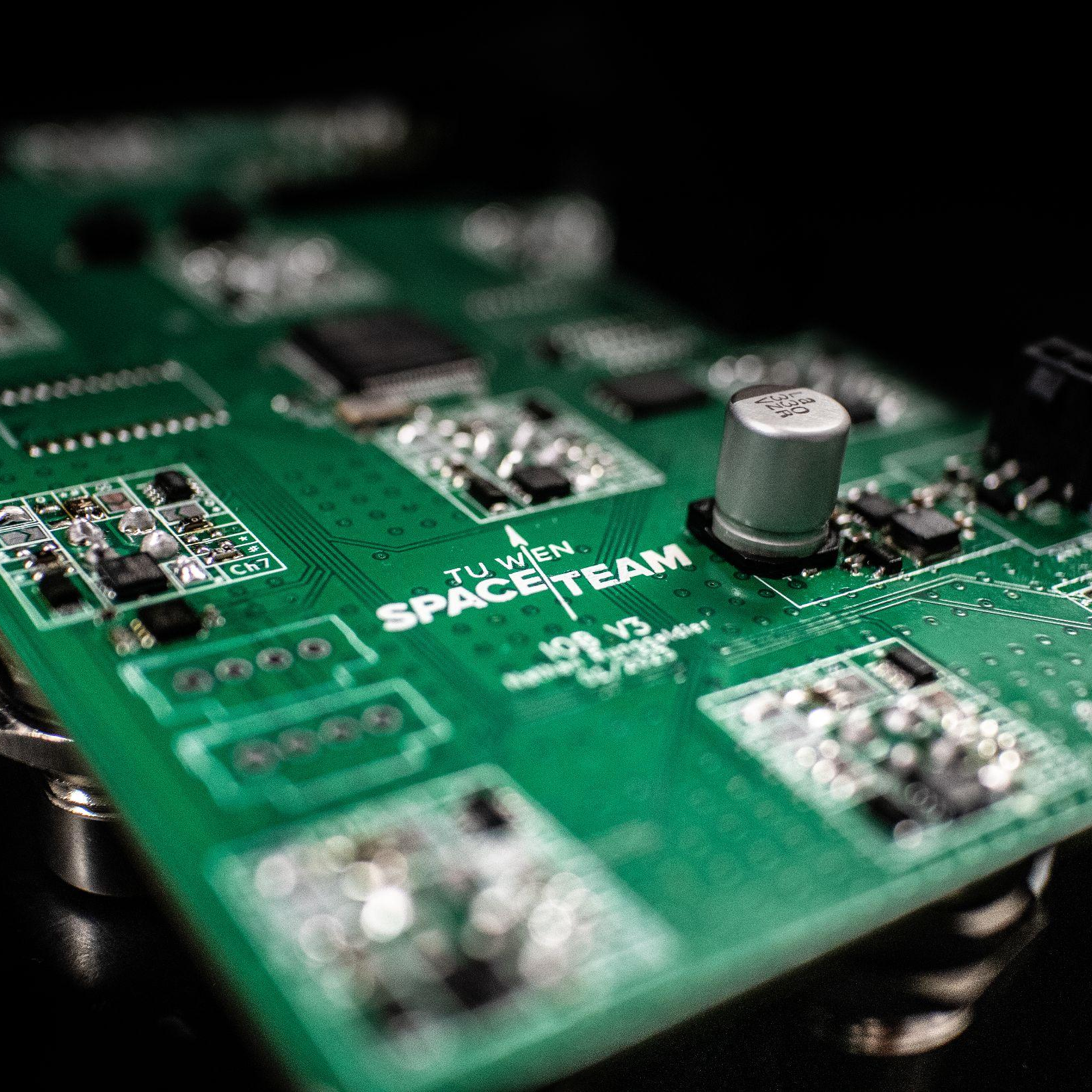
Ground Support Equipment
In order to prepare our rocket for launch and communicate with it during the flight, various systems are being developed. The tanking system efficiently fills the vehicle with oxidizer and pressurant without manual intervention, ensuring safety by avoiding personnel near the pressurized vehicle. A single 300 bar nitrogen bottle pressurizes all systems, with different pressures for tanking and dewar pressurization. The launch rail, extended to 9m, comprises aluminum trusses and standard profiles. A hold-down system prevents premature liftoff and serves as a scale to monitor rocket mass during tanking and measures the actual thrust before takeoff.
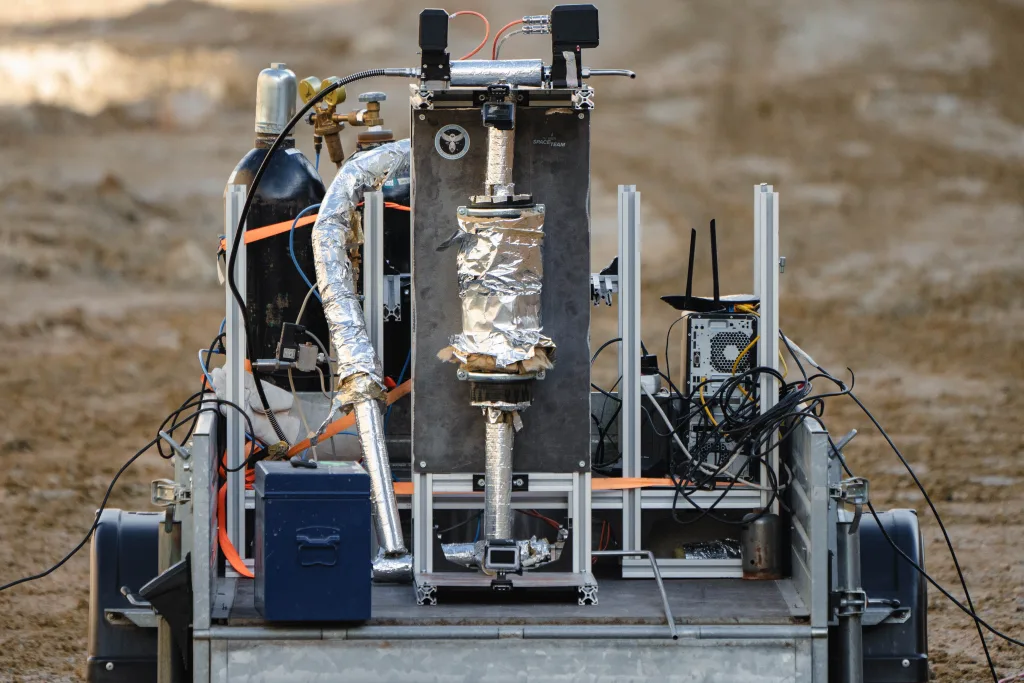